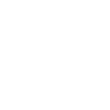
BACKGROUND OF SARCOIDOSIS
Sarcoidosis is a systemic granulomatous disease characterized by a T-helper-1 response with accumulation of CD4+ lymphocytes and activated macrophages in the lungs and affected organs, resulting in characteristic granuloma formation in genetically pre-disposed individuals (Baughman 2003; Baughman 2011). Sarcoidosis most commonly affects young adults of both sexes, although studies have reported more cases in females. The incidence is highest for individuals younger than 40 and peaks in the age-group from 20 to 29 years; a second peak is observed for women over 50 (Nunes 2007). Sarcoidosis occurs throughout the world in all races with an average incidence of 16.5 per 100,000 in men and 19 per 100,000 in women. The disease is most common in Northern European countries and the highest annual incidence of 60 per 100,000 is found in Sweden and Iceland. In the United Kingdom the prevalence is 16 in 100,000. In the United States, sarcoidosis is more common in people of African American descent than Caucasians, with annual incidences reported as 35.5 and10.9 per 100,000, respectively (Henke 1986).
The etiology of this disease is still unclear (Moller 2017). It is hypothesized that one
or more unidentified antigens induce an immune response mediated by alveolar
macrophages and lymphocytes. Due to similarities with granulomas formed
during mycobacterial infections, it is hypothesized that DNA containing complexes originating from a variety of bacteria may be one of these potential antigens
(Eishi 2002; Gupta 2007; Drake 2013). There is evidence of the presence of microbial DNA from mycobacteria, propionibacteria and borrelia in sarcoidosis tissues
suggesting they may participate in sarcoidosis granuloma formation (Chen and
Moller 2014). Bacterial amyloid curli may act as a carrier for such DNA to elicit an
autoimmune response (Tursi 2017; Nicastro and Tukel 2019). Serum amyloid A
derived from the host may participate in the granuloma formation by regulating
inflammation through Toll-like receptor-2 (TLR2) (Chen 2010). Serum amyloid A
localized to macrophages and giant cells within sarcoidosis granulomas but
correlated with CD3(+) lymphocytes, linking expression to local Th1 responses
(Chen 2010). Serum amyloid A activated nuclear factor kappa-light-chain-enhancer of activated B cells (NF-kappaβ) in TLR2-expressing human cell lines, regulated experimental Th1-mediated granulomatous inflammation through IFN-γ, TNF, IL-10, and TLR-2; and stimulated production of tumor necrosis factor, IL-10, and IL-18 in lung cells from patients with sarcoidosis, effects inhibited by blocking TLR-2 (Chen 2010). A significant association was found between TLR9 expression and CD4+ lymphocytes in bronchoalveolar lavage (BAL) of patients with sarcoidosis. Increased TLR9 expression in alveolar macrophages derived from patients with sarcoidosis was also reported and these cells secreted higher levels of cytokines in response to in vitro stimulation (Inaoka 2019). Additional antigens implicated in initiating the inflammatory cascade leading to granuloma formation include beta-actin, intermediate filament vimentin and pathogen-associated molecular patterns (PAMPs) (Cooke 2013; Mortaz 2017).
The granuloma in sarcoidosis is characterized by a core of monocyte-derived epithelioid histiocytes and multinucleate giant cells with interspersed CD4+ T lymphocytes (Iannuzzi 2007). A minority of cells in or near the granuloma are CD8+ T lymphocytes, fibroblasts, regulatory T cells, and B lymphocytes. The T-cell response is biased toward a Th1 phenotype, with important roles for IFN-γ and IL-12 (Moller 1996). Increased expression of key inflammatory mediators, TNF, IFN-γ, IL-2, IL-10, IL-12, IL-18 and transcription factor STAT-1, is indicative of a Th1 mediated immune response (Baughman 2011). More recently, studies have found upregulation of Th17 immune responses producing IL17 and Th17.1 responses expressing INFg in sarcoidosis (Facco 2011; Ramstein 2016). Several studies indicate an expansion of regulatory T cells but with reduced functional capabilities that may be ineffective in suppressing the local exaggerated T cell mediated inflammatory tissue responses (Miyara 2006; Taflin 2009). Sarcoidosis has paradoxical effects on inflammatory processes, characterized by increased macrophage and CD4 helper T cell activation, resulting in accelerated inflammation, but immune response to antigen challenges such as tuberculin is suppressed in the peripheral blood and skin. This response may be mediated by upregulation of the immune checkpoint inhibitor Programmed Death-1 (PD-1) on peripheral blood CD4 T cells in sarcoidosis patients (Celada 2017).
CURRENT TREATMENTS AND STANDARD OF CARE
The natural course of the disease and prognosis are variable from asymptomatic, spontaneous remission over many months to progressive disease requiring treatment (Grunewald 2019). Corticosteroids are recommended as first-line therapy, but an optimal regimen and duration of treatment is not well established. Once treatment is initiated, tapering therapy can be problematic with recurrent inflammation and functional declines. Treatment may differ based on severity of disease, extrapulmonary involvement, as well as physician and patient preferences. Corticosteroid use is effective as an initial therapy but is associated with significant side effects which can impair patients’ quality of life (Judson 2015). Many of the toxicities of corticosteroids are the cumulative side effects such as weight gain, diabetes, osteoporosis, cataracts, and skin fragility (Judson 2015).
Steroid sparing strategies involve immunomodulating drugs that have their own individual drawbacks and toxicities. Agents in common use include methotrexate, leflunomide, azathioprine, mycophenolate, hydroxychloroquine, and infliximab (Ussavarungsi and Gerke 2019).
Methotrexate is often considered as an initial steroid sparing therapy in those who do not have contraindications to its use. Long-term treatment with methotrexate is associated with liver damage in about 10% of people and hence may be a significant concern in people with liver involvement and requires regular liver function test monitoring (Nunes 2007). Methotrexate can also lead to pulmonary toxicity, although this is fairly uncommon. More commonly it can confound the leukopenia caused by sarcoidosis (Nunes 2007). Due to these safety concerns it is recommended that methotrexate is used with folic acid in order to reduce toxicity (Nunes 2007). Azathioprine treatment less frequently can lead to liver damage but carries a higher rate of infectious complications (Baughman 2013; Vorselaars 2013). Leflunomide is sometimes being used as a replacement for methotrexate, possibly due to its purportedly lower rate of pulmonary toxicity (Baughman 2013). Mycophenolic acid has been used successfully for pulmonary sarcoidosis (Brill 2013). Anti-TNF agent infliximab has shown marginal benefit in pulmonary sarcoidosis but may be more effective in non-pulmonary sarcoidosis (Baughman 2011; Judson 2014). The anti-TNF agent adalimumab has also been used as a steroid sparing therapy supported by small anecdotal case series.
RATIONALE FOR THE USE OF SM001 AND PROPOSED MECHANISM OF ACTION
Deoxyribonuclease (DNase1) is a natural occurring enzyme that selectively hydrolyzes single and double stranded DNA. A recombinant human DNase1, dornase alfa, is produced in Chinese Hamster Ovary (CHO) cells and was originally marketed as Pulmozyme®. It is approved for the treatment of patients with cystic fibrosis. In patients with cystic fibrosis this product acts primarily as a mucolytic. However, evidence suggests that it also acts on bacterial DNA to reduce recurrent pseudomonas infections from bacteria found in a biofilm within a patient’s lung (Tetz 2009).
The following describes the mechanism of action (MoA) of DNase1 in pDNA/TLR9 dependent inflammatory and fibrotic disease. Based on this MoA, there is the expectation that DNase1 will reduce pro-inflammatory and pro-fibrotic mediators and, as a result, inhibit progression of inflammation and fibrosis; will reduce or resolve pre-existing inflammation; and will not resolve pre-existing fibrosis in animal models or patients with fibrotic diseases.
Mechanism of action of DNAse1 in pDNA/TLR9 dependent inflammatory and fibrotic disease
At a molecular level, DNAse1 cleaves DNA into small oligonucleotide sequences:
1- If the DNA is eukaryotic in origin and/or prokaryotic in origin, this results in a reduction in viscosity in the medium in which the DNA is found. This is the MoA of the current approved use of DNAse1 in cystic fibrosis.
2- If the DNA is prokaryotic in origin (pDNA), and therefore has hypomethylated CpG sequences, the pDNA, once cleaved by DNAse1 cannot upregulate TLR9. Consequently, TLR9 does not upregulate pro-inflammatory and pro-fibrotic mediators. This may affect the disease progression of pulmonary sarcoidosis and related conditions.
As per Figure 1, the two sources of pDNA in a patient are bacterial (btDNA) and mitochondrial (mtDNA). Mitochondria are the descendants of bacteria internalized into a eukaryotic cell (Cardon 1994).
When bacteria or mitochondria are damaged or lyse, btDNA and mtDNA are released. The causes of these events include:
- Normal bacterial cell death.
- Bacterial death due to host immune responses to infection (e.g. pediatric sepsis ) (Di Caro 2016).
- Patient cell damage due to physical trauma (e.g. ventilation) (Zhang 2010), or chemical trauma (e.g. paraquot or bleomycin) (Li 2015).
- Aging results in increases in mtDNA (Pinti 2014).
All of these events lead to increased btDNA and/or increased mtDNA levels. Increased mtDNA levels have been implicated in many diseases (Boyapati 2017, Collins 2004) and animal models of disease (e.g. sterile and infectious systemic inflammatory response syndrome in non-human primates) (Sursal 2013). In a study examining mtDNA in rheumatoid arthritis patients, PCR‐amplifiable mtDNA was detected in 38 of 54 (70%) of the samples from rheumatoid arthritis patients and in 0 of 17 (0%) of the control patients (Collins 2004). Measurement of mtDNA copy number demonstrated that lepromatous leprosy patients had a significantly higher mtDNA content than control patients (p = 0.008) (Wang 2012). Increased pDNA levels result in increased TLR9 activation (West 2011), which increases pro inflammatory and pro fibrotic mediators. This, in turn, results in the condition or disease noted, such as cardiovascular disease (Nakayama 2018), granulosis with polyangitis (Surmiak 2015), erythema nodosum leprosum when compared with nonreactional lepromatous patients (Dias 2016), and others (Boyapati 2017). In animal models employing lipopolysaccharide (LPS) to mimic sepsis, LPS-induced mtDNA release occurs in a TLR4-dependent manner, and mtDNA causes acute lung injury and systemic inflammation in a TLR9-dependent and TLR4-independent manner (Zhang 2016). In animal models using bleomycin or paraquot to induce inflammation and lung damage as a result of subsequent elevated mtDNA levels, systemically delivered DNAse1 delivered prophylactically reduces mtDNA levels and subsequently reduces inflammation and fibrosis (Li 2015). In human umbilical vein endothelial cells, lysosomal DNAses protect cells against inflammation from mtDNA damage induced by oxidized-low density lipoproteins (Ding 2013). Small interfering RNA (siRNA) knockdown of DNase2 amplifies the mtDNA/TLR9 mediated inflammatory response (Ding 2013). It is likely that the specific condition is related to the specific trigger event that leads to the bacterial or mitochondrial damage.
A number of conditions and infections have been implicated in sarcoidosis, including bacterial infections such as Propionibacterium and Mycobacterium (Eishi 2002), and these have been shown to result in upregulation of TLR9 (Dalpke 2006). Streptococcus pneumoniae exposed to human alveolar epithelial cells in vitro causes the release of mtDNA (Nerlich 2018). mtDNA has also been used to directly induce lung damage in animal models via TLR9 activation (Zhang 2016, Zhang 2014, Kuck 2015). In lung damage murine models induced by mtDNA, treatment with systemically delivered DNAse1 reduces lung epithelial permeability damage (Kuck 2015).
A number of animal models have been developed that reflect parts of the sarcoidosis pathology (Hu 2017). Investigations have revealed that bacteria upregulate TLR9 in HEK-TLR9 cells, including (from high to low upregulation), P. aeruginosa, M. tuberculosis, K. pneumoniae, and P. acnes (Dalpke 2006). This has led to animal models being developed using Propionibacterium to induce inflammation, granulomas, and fibrosis (Jiang 2016, Iio 2010), demonstrate TLR9 upregulation (McCaskill 2006), and demonstrate the lack of ability of Propionibacterium to induce inflammation and granulomatosis in TLR9 -/- mutant murine models (Kalis 2005, Tchaptchet 2012). DNA from M. tuberculosis induces pro-inflammatory cytokine responses through a TLR9 dependent pathway in murine models of TLR9 +/+ and TLR9 -/- mutants (Bafica 2005). Similar animal models have been developed using P. aeruginosa. In murine models employing P. aeruginosa, which upregulates TLR9 to a greater degree than M. tuberculosis and P. acne (Dalpke 2006), treatment with systemically delivered DNAse1 reduces lung epithelial permeability damage (Kuck 2015). Granulomatosis is suppressed in other infectious disease models of TLR -/- mutant mice including Stachybotrys chartarum (a fungus) induced hypersensitivity pneumonitis (Bhan 2013).
It has been demonstrated that TLR9 is expressed in alveolar macrophages from patients with sarcoidosis with chest X-ray types I and II (Schnerch 2016). These TLR9 receptors are fully active, as these cells react to the TLR9 ligand CpG. TLR9 was found to contribute to the immunopathogenesis of sarcoidosis via induction of C-X-C motif chemokine 10 release in the alveolar macrophages (Schnerch 2016). Upregulation in TLR9 mRNA expression was detected in 14 out of 14 patients with pulmonary sarcoidosis (p = 0.05), when compared to 10 control patients (Margaritopoulos, 2010). In animal models of P. acne in mice that are TLR9-/-, which genetically reduces TLR9 levels, lung inflammation and granulosis are inhibited as compared to control wild type mice (Tchaptchet 2016). In animal models in mice that are either TLR9-/-, or TLR9+/+, TLR9-/- mice challenged with mtDNA had no inflammatory response, while TLR9+/+ mice had significant upregulation of inflammatory mediators (Knuefermann 2007). The cleaving of pDNA by systemically delivered DNAse1 may therefore result in the amelioration of a pDNA/TLR9 mediated condition, including pulmonary sarcoidosis, by reducing the upregulation of TLR9.
In a mouse model of pulmonary sarcoidosis using P. acne, inhaled recombinant human DNase1 was shown to markedly attenuate the inflammatory pathological processes leading to granuloma formation (IPST Study Number: 20181114-1, for more details please refer to Section 2.3.1). This model is believed to mimic the human pathological process of pulmonary sarcoidosis through initiation of granuloma formation via a bacterium found in lungs of patients with pulmonary sarcoidosis as outlined above. By extension through the same mechanism of DNase activity, SM001 is expected to degrade the pDNA in lungs of patients with pulmonary sarcoidosis, which could then reduce or eliminate the antigen responsible for the persistent immunological stimulation causing granuloma formation.
Of note, a subset of pulmonary sarcoidosis patients has elevated levels of beta-actin in bronchoalveolar lavage fluid, which may act as an antigen to increase the risk of developing pulmonary fibrosis (Cooke et al. 2013) SM001 will have mucolytic activity on sputum containing extensive DNA from either bacterial or human cell origin and would improve clearance of thick sputum for patients with pulmonary sarcoidosis with that condition.
SUMMARY
There is no curative therapy for sarcoidosis. Although sarcoidosis may resolve on its own, in 30-50% of patients the clinical course is chronic leading to significant burden on patients, negatively effects patients’ quality of life and causing long-term complications.
Sarcoidosis is considered a rare disease by standards of the National Institutes of Health and, according to the American Thoracic Society, there is a lack of large, well designed sarcoidosis studies to guide treatment. Corticosteroid therapy is the routine therapy for patients with symptomatic and progressive pulmonary sarcoidosis. However, the benefits of systemic corticosteroid therapy for pulmonary sarcoidosis patients are limited. Corticosteroid use is quite effective as initial therapy but is invariably associated with significant side effects with chronic use which can impair patients’ quality of life. Steroid sparing strategies involve immunomodulating drugs that have their own considerable potential toxicities, expense and adverse effects. Thus, there is a critical unmet medical need to develop effective, safer treatments for patients with pulmonary sarcoidosis.
Given the significant drawbacks of all currently recommended treatments for sarcoidosis, an effective therapy that targets the inflammatory processes ongoing in the lungs of patients with pulmonary sarcoidosis and that has a reduced adverse effect profile compared with current therapies would be of major benefit and impact to the sarcoidosis community.